Anti-diabetic effect of Daldinia eschscholtzii and Phomopsis mangiferae isolated from Bryophyllum pinnatum
Abstract
The aim of the present study was to isolate endophytic fungi and examine their anti-diabetic as well as antioxidant potential. Twenty-nine endophytic fungi were isolated from the stem and root parts of Bryophyllum pinnatum. Based on the presence of phytoconstituents, 16 endophytic isolates were tested for the inhibition of α-amylase enzyme and free radicals. Thirteen isolates showed significant inhibition of α-amylase enzyme (18.8 ± 0.8 to 62.5 ± 0.4%) and free radicals (15.3 ± 0.2 to 87.4 ± 0.8%). Good inhibitions were found in six isolates, which was further tested to validates its anti-diabetic activity by glucose uptake in yeast cell and glucose diffusion inhibition studies. The most active isolates were identified as Daldinia eschscholtzii (BPS17) and Phomopsis mangiferae (BPK9) by microscopic and molecular identification method. Identified fungal isolates were tested for glucose uptake using 3T3 cell lines (100 µg/mL) and the result showed effective glucose utilization. These finding provides evidence that D. eschscholtzii and P. mangiferae are potent endophytic fungi having anti-diabetic activity.
Introduction
Diabetes mellitus is considered as a chronic metabolic disorder characterized by hyperglycemia which results in causing various chronic vascular complications. One therapeutic strategy to control hyperglycemia is by inhibiting α-amylase, an enzyme involves in the metabolism of carbohydrates where starch will hydrolyze into simple sugars which then enters the bloodstream. Therefore, the level of postprandial blood glucose will be reduced. Commercially available inhibitors are acarbose and miglitol, which competitively and reversibly inhibit these enzymes present in the intestine and pancreas, but the use of synthetic drugs results in causing several adverse effects (Pavithra et al., 2014).
Despite the treatment options available today, many herbal based products are suggested for treating diabetes. Plants such as Branchylaena elliptica (Sagbo et al., 2018), Calpurnia aurea (Belayneh and Birru, 2018), Kandelia candel (Shettar and Vedamurthy, 2017), Momardica charantia (Joseph and Jini, 2013), Punica granatum (Pottathil et al., 2020), Rhizophora apiculata (Shettar and Vedamurthy, 2017), Iphiona aucheri (Shah et al., 2020), Sorghum halepense (Shah et al., 2019), Treculia africana (Ogbonnia et al., 2008) show anti-diabetic activity.
Plants used as traditional medicine that produce strains of endophytes have become an excellent source for finding new bioactive metabolites since their beneficial properties for plants can be a result of metabolites produced by the endophytes (Venkateswarulu et al., 2018). Anti-diabetic effect of the endophytic fungi like Schizophyllum commune, Alternaria sp., Aspergillus sp. and Talaromyces sp. (Kaur, 2018; Rustamova et al., 2020; Tejaswini et al., 2021), Stemphylium globuliferum (Pavithra et al., 2014), Penicillium brevicaule alba Thom. (Gulyamova et al., 2020), Curvularia lunata (Jayant and Vijayakumar, 2021), Cochliobolus sp. (Shoba and Sathiavelu, 2018) have been reported.
Bryophyllum pinnatum (Lam.) Kurz (Syn. Kalanchoe pinnata) belongs to the Crassulaceae family and common names include love plants, miracle leaves and Cathedral bells. This plants B. pinnatum has anti-diabetic (Ojewole, 2005; Ogbonnia et al., 2008; Aransiola et al., 2014; Ezeagu et al., 2017; Ojo et al., 2018) and antioxidant effects (Gupta et al., 2016; Mbachu et al., 2019; Daniel et al., 2020). The anti-diabetic activity was reported in B. crenata (Effah-Yeboah et al., 2021) and B. laciniata (Fernandes et al., 2019).
Three biologically active phenolic compounds namely bryophillor, bryophorone and bryopholenone were isolated from B. pinnatum and these biologically active molecules have antioxidant properties (Gupta et al., 2016). Alpha glycosidase activity was exhibited by two flavonoid glycosides [quercetin 3-O-α-L-rhamnoside and quercetin-3-O-α-L-arabinopyranosyl (1 → 2)α-L-rhamnopyranoside ] isolated from leaves ethyl acetate extract of B. pinnatum (Sofa et al., 2018).
The endophytes isolated from the plant B. pinnatum were Aspergillus brunneoviolaceus (Okonkwo-Uzor et al., 2022), Diaporthe phaseolorum (Sowemimo et al., 2008, Ali et al., 2023).
Cytochalasin D and (3R,4R)-3,4,5-trihydroxy-1-tetralone isolated from Diaporthe phaseolorum of B. pinnatum leaf had shown cytotoxic activity (Sowemimo et al., 2008, Ali et al., 2023). Anti-diabetic and antioxidant activity were exhibited by unknown endophytic fungi isolated from the genus Bryophyllum (Kaur, 2018). Therefore, the current study focused on the isolation and identification of endophytic fungi from stem and root parts of B. pinnatum and examines their anti-diabetic and antioxidant effects.
Materials and Methods
Collection of plant samples
The plant samples were collected from the Vellore Institute of Technology, Vellore, Tamil Nadu, India. Plant materials were transferred to the laboratory in a sterilized bag and processed within 24 hours of sampling (Bhardwaj et al., 2015).
Isolation of endophytic fungi
Healthy stem and root samples are thoroughly washed in running tap water to remove dust and debris and then surfaces sterilized according to the method described elsewhere with slight modification. Soak the plant segments in 95% ethanol for 30 sec, 4% sodium hypochlorite for 3 min, and 95% ethanol for 30 sec and then washed 3 times in sterilized distilled water and dried with sterilized blotting paper in sterile condition. After drying, cut each segments into small pieces and place them on potato dextrose agar plate and incubated at 27 ± 2°C for 1 week. Monitor the growth of endophytic colonies every day. The fungi grown from the samples were then transferred to fresh potato dextrose agar plate plates to isolate pure colonies and maintained by subsequent subculturing method (Bhardwaj et al., 2015).
Secondary metabolite production
Mycelium from the edge of the growing colony (agar block) was inoculated in potato dextrose broth and incubated for 21 days at 27 ± 2°C for fungal metabolite production by the fermentation method. After incubation period, each culture was filtered by using Whatman No. 1 filter paper to separate fungal filtrate and mycelium mat. A solvent extraction procedure was carried out for the fungal filtrate using dichloromethane, ethyl acetate and butanol and mycelium mat in methanol for the extraction of fungal crude. An equal amount of solvents were added to the fungal filtrate in separatory funnel and shaken vigorously for 10 min and kept aside until two transparent immiscible layers were formed. The solvent containing the extracted compounds were collected and evaporated to yield dry crude. The obtained fungal crudes were used to carry out the further studies (Jayant and Vijayakumar, 2021).
Morphological and molecular identification of endophytic fungi
Lactophenol cotton blue method was used to identify the morphological observation of endophytic fungi by the method reported elsewhere (Kumari and Silva, 2017). For molecular identification, about 100 mg of fungal mycelium was taken for DNA isolation. DNA was isolated according to the standard method described elsewhere (Ferdous et al., 2022; Hamzah et al., 2018). Nuclear ribosomal internal transcribed spacer (ITS) of the fungal isolate was amplified with a forward primer, ITS1 (5Ì-TCCGTAGGTGAACCTGCGG-3Ì) and reverse primer, ITS4 (5Ì-TCCTCCGCTTATTGATATGC-3Ì) (White et al., 1990). The final volume of the reaction mixture 25 µL (1.5 µL of forward and reverse primer, 5 µL of deionized water and 12 µL of Taq Master Mix) was added to 1.5 µL of DNA isolates. For negative control, DNA was replaced with distilled water to ensure that there was no contamination. PCR was programmed and performed by thermal cycling conditions. The millipore mounting kit was used to remove unincorporated PCR primers and dNTP from the PCR product. Sequencing was carried out for the primers obtained from PCR products and reaction was performed using ABI PRISM® BigDyeTM terminator cycle sequencing kit and AmpliTaqTM DNA polymerase. The resulting endophytic fungi sequence was then exposed to the nucleotide BLAST search and similar regions of query sequence were compared with biological sequence deposited in NCBI database. To understand evolutionary relationships, phylogenetic tree was constructed for each isolate strain using the MUSCLE 3.7 program. Phylogenetic tree was built using PhyML software (Ferdous et al., 2022).
Qualitative phytochemical analysis
Qualitative phytochemical screening was performed for the fungal extracts by following the procedure described elsewhere (Bhardwaj et al., 2015). The fungal crude extracts were analysed for the presence of phenol, flavonoids, alkaloids, terpenoids, steroids, saponin and tannin.
Box I: Antioxidant Activity by DPPH Assay
1,1 Diphenyl 2-picryl hydrayzl are dark purple, long-lived organic nitrogen radicals. When DPPH solution is mixed with an antioxidant, it changes color from purple to yellow from the corresponding hydrazine.
Requirements
Ascorbic acid, DPPH (1,1-diphenyl-2-picryhydrazyl), Fungal crude extracts, Methanol
Procedure
Step 1: DPPH was prepared by dissolving in methanol for making stock solution
Step 2: Add 2 mL of DPPH solution and different concentration (50, 100, and 150 µg/mL) of crude extracts in test tube. Incubate for 30 min under complete darkness
Step 3: Absorbance was taken at 517 nm.
Calculation
The ability of the fungal crude extracts to scavenge DPPH free radicals was calculated by using the following equation,
Percentage inhibition of free radicals = Absorbance of control - Absorbance of sample/Absorbance of control × 100
References
Xiao et al., 2020; Baliyan et al., 2022
In vitro anti-diabetic activities
Alpha-amylase inhibition assay
About 1 mg of fungal crude extracts was prepared by dissolving in solvents. Alpha amylase enzyme was slowly dissolved in 0.1 M of phosphate buffer. In each test tube, add 100 μL of solution (enzyme+ buffer) and 100 μL of samples of varying concentrations (100, 200 and 300 μg/mL) followed by addition of 100 μL of 1% starch solution and incubated at 37ºC for 10 min. After the incubation time, add 200 μL of DNSA (3,5-dinitrosalicylic acid) solution and kept in boiling water bath for 10 min. Cool down to room temperature, add 200 μL of 40% Rochelle salt to the mixture and diluted with distilled water, absorbance was taken at 540 nm. Acarbose was used as the standard (Tiwari et al., 2017). The percentage inhibition of alpha-amylase was calculated using the formula:
% Inhibition of α-amylase = (Abs of control - Abs of sample/Abs of control) × 100
Glucose uptake by yeast cell study
The commercially available baker’s yeast was dissolved in distilled water and kept for overnight incubation. After the incubation period, the tubes were centrifuged (3,000 × g, 5 min) until a clear supernatant was obtained. In the same way, 10% (v/v) yeast suspension was prepared. Different concentrations of crude extracts (100-500 µg/mL) were added in each test tube containing 1 mL glucose solution (10 and 25 mM) and the mixture was incubated at 37ºC for 10 min. After the incubation period, the reaction was initiated by adding 100 µL of yeast suspension, and vortex and incubated at 37ºC for 60 min. After the incubation period, the tubes were centrifuged to evaluate the glucose concentration present in the supernatant by taking the absorbance in UV-Vis spectrophotometer at 520 nm (Paul and Majumdar, 2020). The percentage of glucose uptake by yeast cells was calculated by the following formula:
%Increase in glucose uptake = (Abs of control - Abs of sample/Abs of control) × 100
Glucose diffusion inhibition assay
The extracts (1.1 mL) were taken in the dialysis membrane (12000 MW, Hi Media Laboratories, India) with a glucose solution (0.22 mL in 0.15 M NaCl2). The edges of the membrane were tied and then immersed in a beaker containing 40 mL of 0.15 M sodium chloride solution and 10 mL distilled water. The beakers were placed in a magnetic stirrer and the entire process was carried out in room temperature. Pipette out 4.5 mL of the solution for every 30 min up to 150 min of observation period. Each time the volume was compensated by adding new solution. The absorbance was taken at 248 nm in a UV spectrophotometer. Control tube was also carried out in a same way by replacing sample with distilled water (Jayamol et al., 2020).
Glucose uptake assay by 3T3 cell lines
Cell culture
The 3T3 cell lines were purchased from National Centre for Cell Sciences, Pune, India. Cells were maintained with DMEM containing 10% FBS (fetal bovine serum), penicillin (100 U/mL) and antibiotics (100 µg/mL) in a humidified environment. 3T3 cell lines was cultured with DMEM in 24-well plates at a final volume of 1000 µL with a cell thickness of 6 × 104 cells/wells and incubated for 48 hours until the cells intersected.
Preparation of sample
In a six well plate add 5 mL of solution (cells+ medium) and 1 mL of fungal crude extracts (100 µg/mL) and standard (1 IU/mL) and incubated for 24 hours. After the incubation period, the entire medium was taken off from the 6-well plate and washed with 2 mL of 10× PBS. Then add 150 µL of 10% SDS and shaken well. With the cell scraper, the cells were transferred to the eppendorf tube and named accordingly. Then the tubes were centrifuged and the supernatant was transferred to the marked eppendorf and stored at 4°C for further process.
Determination of glucose concentration by DNS assay
In each test tube add 200, 400, 600, 800, and 1000 µL of glucose stock solution. From each supernatant sample, 100 µL was added and a volume of 2 mL was prepared with distilled water. Distilled water serves as the blank. Add 1 mL of DNS reagent to all the test tubes and cover it with aluminium foil and kept in a boiling water bath for 5 min at 100°C to observe the discoloration. The test tubes were allowed to cool down and absorbance was taken at 540 nm in different time intervals. The graph was plotted with the amount of glucose (mg/mL) on the X-axis and the absorbance value of the sample on the Y-axis. Measure the absorbance of the sample and the unknown concentration was detected from the standard (Kumar et al., 2021).
GC-MS analysis
The extract was analysed with Perkin Elmer Clarus 600 mass spectrometer (EI) with an Elite-5MS capillary column (30, 0.5 mm ID, 259 m df), the device was stored for 6 min at an initial temperature of 55° C in the oven for 3 min and the ramp program for 10 min-1 to 300°C at a constant flow rate of 1 mL/min, helium was used as a carrier gas, the base temperature and mass transfer line was set at 240°C. Turbo software version 5.4.2 was used for spectral analysis. Individual components recognized in the general mass spectrum by the National Institute of Standards and Technology (NIST-LIB 0.5) included in the GC-MS system software (Wiley GC-MS-2007) and literature data. Metabolites present in the fungal crude extract are separated by a gas chromatography column. Each compound separated by GC enters the MS and get ionized. The MS ionization spectrum was recorded and compared with the mass spectrum of compounds known in the NIST library. The MS spectrum accurately maps the molecular weight of each molecule (Shankar et al., 2018).
In vitro antioxidant assay
The ability of the fungal crude extracts to inhibit DPPH free radicals was assessed using standard methods. Crude extracts were taken in a test tube at different concentrations (50, 100, and 150 µg/mL) and 2 mL of DPPH was added. The tubes were incubated for 30 min at room temperature under dark condition and after the incubation time, the absorbance was taken at 517 nm in UV-Vis spectrophotometer. Ascorbic acid was used as the standard (Gurnani et al., 2016). The percentage inhibition of free radicals was calculated using the following formula:
% Inhibition of free radicals = [Abs of control - Abs of sample/Abs of control] × 100
Statistical analysis
The experiments were conducted in triplicates and the results are expressed in mean ± SEM. Graph pad prism software was used for statistical calculation.
Results
Isolation of endophytic fungi and extraction of secondary metabolites
Twenty-nine pure endophytic fungi were successfully isolated from the sterile stem and root segments of B. pinnatum (Figure 1). All the fungal endophytes were subcultured on new agar plates and stored in various ways, such as agar plates, and agar slants. Twenty-nine endophytic fungi were grown separately and on a large scale in potato dextrose broth to extract secondary metabolites by using different polarities of solvent such as dichloromethane, ethyl acetate, butanol and fungal mat in methanol by the solvent extraction process. The obtained fungal extracts were dried and stored at 4°C for further studies.
Figure 1: Colony morphology of endophytic fungi isolated from stem and root parts of Bryophyllum pinnatum
Qualitative phytochemical analysis of fungal crude extracts
Qualitative phytochemical analyses were performed for ethyl acetate and methanol fractions of twenty-nine endophytic fungi isolates. The presences of phytoconstituents in endophytic fungi are responsible for the various biological activities shown by the crude extracts and the use of this plant as traditional medicine prac-tices since ancient times. Based on the presence of phytoconstituents, 16 endophytic fungi were further selected to examine antioxidant and anti-diabetic potential (data not shown).
Anti-diabetic activity
Alpha amylase inhibition assay
Alpha amylase inhibition assay was performed to determine the anti-diabetic activity of fungal crude extracts. Thirteen endophytic fungi isolate showed inhibition of alpha amylase which was compared with the standard drug acarbose. The tested extracts showed significant inhibition of about 18.7 ± 0.7%- 62.5 ± 0.3% and the standard (acarbose) showed 70.5 ± 0.5% (Table I). The maximum inhibition was showed by BPS17 and BPK9 of about 61.7 ± 1.7% in dichloromethane extract and 62.5 ± 0.3% in ethyl acetate extract respectively. The least inhibition was exhibited by BPR1 isolate of about 18.7 ± 0.7% in 300 µg/mL concentration. Based on the result obtained in antioxidant and α-amylase inhibition studies six isolates namely BPS4, BPS7, BPS17, BPR7, BPK9 and BPR10 were further chosen to validate its anti-diabetic activity by glucose uptake by yeast cell and glucose diffusion inhibition studies.
Table I: Alpha-amylase inhibition activity of fungal extracts obtained from stem and root endophytic fungi
Fungal extracts (μg/mL) | %Inhibition of α-amylase | ||||
---|---|---|---|---|---|
Dichloromethane | Ethyl acetate | n-Butanol | Methanol | ||
BPS4 | 100 | 15.3 ± 1.0 | 23.2 ± 0.9 | 21.2 ± 0.7 | 28.2 ± 0.6 |
200 | 39.1 ± 0.6 | 46.8 ± 0.8 | 28.6 ± 0.6 | 26.2 ± 0.3 | |
300 | 46.8 ± 0.8 | 57.2 ± 0.4 | 32.26 ± 0.6 | 31.8 ± 0.7 | |
BPS6 | 100 | 23.9 ± 0.5 | 25.9 ± 0.9 | 18.82 ± 0.7 | 17.3 ± 1.0 |
200 | 27.2 ± 0.8 | 35.1 ± 0.7 | 26.63 ± 0.4 | 23.9 ± 0.5 | |
300 | 32.6 ± 0.8 | 38.6 ± 0.6 | 29.25 ± 0.7 | 28.9 ± 0.7 | |
BPS7 | 100 | 28.2 ± 1.2 | 29.2 ± 0.5 | 25.6 ± 0.2 | 27.7 ± 0.6 |
200 | 33.8 ± 1.1 | 45.6 ± 0.7 | 28.3 ± 0.4 | 35.2 ± 0.8 | |
300 | 37.2 ± 1.6 | 52.3 ± 0.1 | 33.8 ± 0.6 | 38.5 ± 0.6 | |
BPS9 | 100 | 11.6 ± 0.2 | 19.5 ± 0.8 | 17.8 ± 0.3 | 13.3 ± 0.8 |
200 | 17.3 ± 0.6 | 35.4 ± 0.3 | 22.5 ± 0.8 | 20.7 ± 0.3 | |
300 | 23.8 ± 0.7 | 42.4 ± 0.2 | 25.2 ± 0.9 | 27.9 ± 0.7 | |
BPS12 | 100 | 12.3 ± 0.6 | 17.2 ± 0.4 | 15.15 ± 0.6 | 17.3 ± 0.8 |
200 | 16.2 ± 0.7 | 21.7 ± 0.6 | 26.5 ± 0.6 | 23.6 ± 0.6 | |
300 | 22.7 ± 0.8 | 28.8 ± 0.7 | 31.3 ± 0.7 | 28.8 ± 0.7 | |
BPS13 | 100 | 20.6 ± 0.3 | 29.0 ± 0.8 | 27.1 ± 0.8 | 18.7 ± 0.2 |
200 | 27.5 ± 0.5 | 36.3 ± 0.2 | 38.2 ± 0.7 | 25.1 ± 0.7 | |
300 | 33.3 ± 0.7 | 42.2 ± 0.3 | 58.2 ± 0.3 | 28.5 ± 0.3 | |
BPS17 | 100 | 31.1 ± 1.0 | 29.8 ± 0.5 | 27.2 ± 0.7 | 25.7 ± 0.4 |
200 | 47.4 ± 1.3 | 37.6 ± 0.4 | 36.3 ± 0.6 | 33.4 ± 0.8 | |
300 | 61.8 ± 1.7 | 45.2 ± 0.2 | 43.6 ± 0.3 | 41.4 ± 0.8 | |
BPR1 | 100 | 10.2 ± 0.5 | 20.3 ± 0.3 | 16.8 ± 0.6 | 10.8 ± 0.3 |
200 | 13.3 ± 0.3 | 23.6 ± 0.1 | 18.5 ± 0.3 | 13.7 ± 0.2 | |
300 | 18.8 ± 0.8 | 27.8 ± 0.3 | 20.4 ± 0.5 | 19.9 ± 0.9 | |
BPR3 | 100 | 10.2 ± 1.8 | 21.5 ± 0.2 | 12.7 ± 0.7 | 27.9 ± 0.4 |
200 | 15.7 ± 0.5 | 32.2 ± 0.6 | 17.9 ± 0.6 | 39.3 ± 0.1 | |
300 | 23.7 ± 0.6 | 39.5 ± 0.6 | 25.2 ± 0.2 | 59.6 ± 0.4 | |
BPR7 | 100 | 31.3 ± 0.1 | 32.8 ± 0.7 | 28.2 ± 0.5 | 23.7 ± 0.2 |
200 | 45.5 ± 0.7 | 47.2 ± 0.6 | 33.6 ± 0.6 | 35.6 ± 0.2 | |
300 | 51.7 ± 0.8 | 53.3 ± 0.3 | 41.4 ± 0.2 | 38.3 ± 0.4 | |
BPK9 | 100 | 30.2 ± 0.7 | 31.0 ± 0.5 | 24.8 ± 0.4 | 18.9 ± 0.6 |
200 | 39.3 ± 0.2 | 48.1 ± 0.2 | 32.2 ± 0.8 | 26.8 ± 0.2 | |
300 | 46.3 ± 1.3 | 62.5 ± 0.4 | 39.8 ± 0.3 | 34.7 ± 0.3 | |
BPR10 | 100 | 29.9 ± 0.7 | 30.2 ± 0.7 | 26.2 ± 0.3 | 23.9 ± 0.1 |
200 | 42.7 ± 0.3 | 43.5 ± 0.3 | 35.8 ± 0.5 | 37.3 ± 0.5 | |
300 | 47.6 ± 0.3 | 49.7 ± 0.1 | 42.7 ± 0.5 | 46.9 ± 0.6 | |
BPR12 | 100 | 12.9 ± 0.5 | 28.4 ± 0.3 | 13.7 ± 0.2 | 11.7 ± 0.3 |
200 | 18.7 ± 0.7 | 31.8 ± 0.5 | 17.9 ± 0.8 | 16.3 ± 0.4 | |
300 | 25.2 ± 0.7 | 38.5 ± 0.3 | 29.6 ± 0.7 | 24.5 ± 0.5 | |
Standard (acarbose) | 100 | 33.5 ± 0.2 | |||
200 | 50.3 ± 0.2 | ||||
300 | 70.5 ± 0.5 | ||||
Data are mean ± SEM; n = 3 |
Glucose uptake by yeast cell study
The fungal crude extracts (dichloromethane, ethyl acetate, butanol and methanol) obtained from six endophytic fungi were taken at different concentrations to determine the glucose uptake by yeast cell study. After treating yeast cells with fungal crude extracts, dose-dependent glucose uptake was observed in both 10 and 25 mM glucose concentration (Figure 2). As the percentage of glucose concentration increases, the uptake of glucose by yeast cells also increased. The maximum glucose uptake was exhibited by BPS17 and BPK9 of about 70.1 ± 1.8% and 72.3 ± 1.7% respectively in the presence of 25 mM glucose concentration at 500 µg/mL concentration of crude extracts. The standard drug (metformin) had shown 75.1 ± 1.8% of glucose uptake in the presence of 25 mM glucose concentration whereas the glucose uptake was found to be comparatively low in 10 mM glucose concentration of about 63.1 ± 1.9% in 500 µg/mL. The results revealed that the rate of increase in glucose absorption by yeast cells is directly proportional to the concentration of glucose.
Figure 2: Effect of fungal crude extracts on glucose uptake by yeast cells. Data are mean ± SEM; n = 3
Effect of fungal crude extracts on glucose diffusion inhibition study
In this study, dialysis membrane containing glucose and extracts soaked in sodium chloride solution was used to evaluate the inhibition of glucose transfer. Glucose diffuse into the sodium chloride (external) solution was measured at 30, 60, 90, 120 and 150 min and the effect of fungal extracts on glucose diffusion inhibition has been summarized in Table II. All the extracts showed an inhibitory effect whereas dichloromethane extract of BPS17 and ethyl acetate extract of BPK9 maintained the maximum inhibition of glucose diffusion of about 64.2 ± 0.3% and 66.5 ± 0.7% respectively, close to the standard drug (acarbose) of about 71.5 ± 1.2%.
Table II: Effect of fungal crude extracts on glucose diffusion through dialysis membrane
Fungal crude extracts (1 mg/mL) |
%Glucose diffusion inhibition at every 30 min | |||||
---|---|---|---|---|---|---|
30 min | 60 min | 90 min | 120 min | 150 min | ||
BPS4 | Dichloromethane | 41.6 ± 0.5 | 43.9 ± 1.0 | 44.4 ± 0.6 | 50.0 ± 0.4 | 53.3 ± 0.2 |
Ethyl acetate | 42.4 ± 0.9 | 43.8 ± 0.8 | 47.2 ± 1.0 | 50.9 ± 0.6 | 54.1 ± 0.6 | |
n-Butanol | 25.5 ± 0.2 | 26.0 ± 0.3 | 31.3 ± 0.6 | 35.3 ± 0.8 | 37.8 ± 0.7 | |
Methanol | 32.5 ± 0.5 | 36.0 ± 0.6 | 38.6 ± 0.8 | 40.6 ± 0.9 | 46.9 ± 1.1 | |
BPS7 | Dichloromethane | 22.5 ± 0.5 | 25.9 ± 0.6 | 26.4 ± 0.7 | 32.6 ± 0.6 | 36.9 ± 1.0 |
Ethyl acetate | 32.5 ± 1.0 | 36.4 ± 0.8 | 38.2 ± 0.9 | 43.6 ± 0.2 | 45.0 ± 0.5 | |
n-Butanol | 16.0 ± 0.8 | 17.3 ± 1.3 | 21.1 ± 0.6 | 25.6 ± 0.3 | 29.9 ± 0.8 | |
Methanol | 7.4 ± 0.6 | 9.6 ± 0.7 | 15.0 ± 0.6 | 19.8 ± 0.4 | 21.6 ± 1.3 | |
BPS17 | Dichloromethane | 46.8 ± 0.2 | 51.0 ± 0.9 | 55.3 ± 0.8 | 60.9 ± 0.1 | 64.2 ± 0.4 |
Ethyl acetate | 37.7 ± 0.8 | 41.8 ± 0.3 | 45.1 ± 0.4 | 49.6 ± 1.5 | 52.8 ± 0.5 | |
n-Butanol | 24.2 ± 0.9 | 28.0 ± 0.1 | 32.5 ± 0.8 | 37.2 ± 1.1 | 41.7 ± 0.6 | |
Methanol | 29.4 ± 0.6 | 33.5 ± 0.6 | 37.0 ± 0.5 | 41.5 ± 0.2 | 46.1 ± 0.7 | |
BPR7 | Dichloromethane | 14.3 ± 0.2 | 18.4 ± 0.2 | 22.0 ± 0.9 | 26.7 ± 0.4 | 30.6 ± 1.0 |
Ethyl acetate | 19.5 ± 0.4 | 23.0 ± 0.8 | 26.0 ± 0.4 | 29.2 ± 0.2 | 33.9 ± 0.4 | |
n-Butanol | 7.1 ± 0.3 | 12.6 ± 1.3 | 15.5 ± 0.3 | 16.0 ± 0.2 | 16.8 ± 1.5 | |
Methanol | 6.1 ± 0.6 | 10.5 ± 0.3 | 13.8 ± 0.6 | 14.2 ± 0.7 | 14.7 ± 0.6 | |
BPK9 | Dichloromethane | 40.7 ± 0.2 | 43.9 ± 0.9 | 47.6 ± 0.9 | 52.7 ± 0.6 | 56.5 ± 0.4 |
Ethyl acetate | 45.9 ± 0.2 | 49.8 ± 0.3 | 52.8 ± 0.7 | 56.6 ± 0.3 | 66.5 ± 0.8 | |
n-Butanol | 34.6 ± 1.8 | 37.7 ± 0.6 | 39.8 ± 0.6 | 43.4 ± 0.9 | 45.1 ± 1.2 | |
Methanol | 29.9 ± 0.3 | 34.3 ± 0.7 | 38.2 ± 0.6 | 42.3 ± 0.7 | 43.1 ± 0.6 | |
BPR10 | Dichloromethane | 19.5 ± 0.3 | 23.8 ± 0.8 | 26.4 ± 0.8 | 30.6 ± 0.2 | 31.0 ± 0.2 |
Ethyl acetate | 32.0 ± 0.8 | 36.0 ± 0.5 | 39.0 ± 0.7 | 41.6 ± 0.7 | 45.9 ± 0.9 | |
n-Butanol | 7.8 ± 1.1 | 8.9 ± 0.6 | 12.8 ± 1.0 | 17.4 ± 0.8 | 22.5 ± 0.6 | |
Methanol | 16.5 ± 0.7 | 19.7 ± 0.7 | 22.8 ± 1.3 | 27.8 ± 0.6 | 31.7 ± 0.2 | |
Standard (Acarbose) | 48.9 ± 1.2 | 54.4 ± 1.0 | 60.6 ± 0.7 | 67.8 ± 0.8 | 71.6 ± 1.3 | |
Data are mean ± SEM; n = 3 |
Morphological and molecular identification of endophytic fungi
Based on the in vitro antioxidant and anti-diabetic studies, BPS17 and BPK9 are considered as a potent strain and it was identified as Daldinia eschscholtzii and Phomopsis mangiferae respectively based on the microscopic observation by LPCB method (Figure 3A, B, C) and 18s rRNA gene sequencing. The sequence was submitted to GeneBank and the accession number was obtained OP740989 and OP741081 respectively. The phylogenentic relationship was established through homologous nucleotide sequences and evolutionary tree was constructed (Figure 3D, E).
Figure 3: Microphotograph of LCB stain at 100x view of hyphae, conidiophores, conidia of BPS17 (A, B) and BPK9 (C) and phylogenetic tree (D, E) of fungal isolates
Glucose uptake in 3T3 cell lines
D. eschscholtzii and P. mangiferae are considered as the potent endophytic strains, therefore dichloromethane and ethyl acetate extracts were chosen to study the effect of glucose uptake in 3T3 cell lines. The highest glucose uptake activity was found in P. mangiferae as the concentration of glucose decreases from the 0th hour to 24th hour of about 2.5 ± 0.0 to 1.9 ± 0.0 µg, whereas the activity was slightly lower in D. eschscholtzii and the results were compared with the standard anti-diabetic drug, insulin showed the glucose uptake by 3T3 cell lines from 2.3 ± 0.1 to 1.8 ± 0.0 µg of glucose concentration (Figure 4). The study concludes that the uptake of glucose in 3T3 cell lines was found in both P. maniferae and D. eschscholtzii.
Figure 4: Effect of glucose uptake in 3T3 cell lines of BPS17 (dichloromethane) and BPK9 (ethyl acetate) crude extracts. Data are mean ± SEM; n=3
GC-MS analysis of fungal crude extracts
D. eschscholtzii and P. mangiferae were chosen as potent endophytic isolates. Therefore, this analysis was carried out to find out the biologically active compounds present in the dichloromethane extract of BPS17 and ethyl acetate extract of BPK9 using gas chromatography mass spectrometer. Chromatogram obtained from GC-MS analysis (Figure 5). The major compounds found in D. eschscholtzii were indano[2,1-D]1,3-dioxane (M.W - 176), and 1(2H)-naphthalenone, 3,4-dihydro-8-methyl- (M.W - 160) present in the retention time of 15.8 and 15.9 with area percentage of 20.7% and 24.5% respectively. P. mangiferae as shown 3-nonanone, 2-methyl- (M.W - 156) and 1,6-dimethyl-3,7,9-trioxabicyclo(4,2,1)nonane (M.W- 158) as the major compounds with the retention time of 13.9 and 17.1 along with area percentage of 41.5% and 34.1% respectively.
Figure 5: GC-MS chromatogram of fungal crude extracts D. eschscholtzii (BPS17) (A) and P. mangiferae (BPK9) (B)
Antioxidant activity
The reaction is visible when the color changes from purple to yellow. The antioxidant activity was performed for the dichloromethane, ethyl acetate, butanol and methanol extracts of 16 endophytic fungi based on the presence of phytochemical constituents. The results indicate that inhibition percentage increases as the concentration of crude extracts increases. The extracts obtained from thirteen endophytic fungi showed significant inhibition of free radicals (Figure 6), whereas BPS17 and BPK9 had the highest inhibition percentage of free radical of about 87.3 ± 0.8% in dichloromethane extract and 85.1 ± 0.8% in ethyl acetate extract respectively and the unknown sp. (BPR1) showed least antioxidant activity of about 15.3 ± 0.2%. The standard ascorbic acid had 92 ± 0.2% of antioxidant activity.
Figure 6: DPPH free radical scavenging activity of fungal crude extracts. Data are mean ± SEM; n = 3
Discussion
D. eschscholtzii and P. mangiferae are considered as the potent endophytic strains shown good inhibition of alpha amylase enzyme, glucose uptake in yeast cell and 3T3 cell lines, glucose diffusion inhibition study and also in inhibition of free radicals. Twenty-nine endophytic fungi were isolated from stem and root parts of B. Pinnatum, in which BPS17 and BPK9 showed maximum anti-diabetic and antioxidant effects and identified as D. eschscholtzii and P. mangiferae respectively.
A study showed 22 endophytic fungi isolated from Momordica charantia and Trigonella foenum-graceum. Among these, 9 extracts exhibited α-amylase and α-glycosidase inhibition activity. The fungal isolate which exhibited maximum inhibition activity was identified as Trichoderma atroviride and Stemphylium globuliferum (Pavithra et al., 2014).
In the present study, the maximum anti-diabetic activity was reported in dichloromethane extract of D. eschscholtzii isolated from B. pinnatum whereas, the same effect was found in ethyl acetate extract of D. eschscholtzii isolated from Cinnmomum bejolghota (Wutthiwong et al., 2021). Five compounds namely (+)-regiolone (isosclerone), (+)-3,4-dihydro-3,4,8-trihydroxynaphthalenone, (+)-mellein, daldiniaeschsone A and daldiniaeschsone B were isolated from D. eschscholtzii of Cinnamomum bejolghota. And all these compounds exhibited anti-diabetic activity with an IC50 value of 0.16-0.85 mM (Wutthiwong et al., 2021). In this study, the major compound found in dichloromethane extract of D. eschscholtzii are indano[2,1-D]1,3-dioxane, 1(2H)-naphthalenone,3,-dihydro-8-methyl- and O-metyliso-urea hydrogen sulphate whereas the ethyl acetate extract of P. mangiferae contains major compounds such as 3-nonanone,2-methyl- and 1,6-dimethyl-3,7,9-trioxabicyclo(4,2,1)nonane. So, the presences of these compounds are responsible for the maximum anti-diabetic activity showed by endophytic strains of D. eschscholtzii and P. mangiferae. The previous studies as isolated peniorcinols A-C, penialidins A, penialidin F, myxo-trichin C, riboflavin, indole-3-acetic acid and 2-(4-hydroxy-2-methoxy-6-methylphenyl) acetic acid from endophytic fungi, in which peniorcinols C and indole-3-acetic acid has shown good anti-diabetic (Bai et al., 2021). Aspergillus awamori isolated from A. nilotica showed 81% and 80% inhibition of α-amylase and α-glycosidase respectively (Singh and Kaur, 2016). The previous findings reported anti-diabetic activity was exhibited by ethyl acetate extract of Penicillium citrinum, isolated from Gymnema sylvestre (Vidyasagar et al., 2021) and P. lanosum and P. radiatolobatum (Naveen et al., 2023). The current findings reported that ethyl acetate and dichloromethane extracts of P. mangiferae and D. eschscholtzii respectively have showed good inhibition of α-amylase enzyme.
The cell lines are considered as a good models for glucose absorption study, since they are widely used to explain the mechanism of glucose absorption in muscles having a complete insulin signaling pathway and show insulin-sensitive GLUT4. The main glucose transporter presented in skeletal muscle and adipose tissue is GLUT4, which is transferred from the intracellular membrane storage site to the plasma membrane (Das and Devi, 2015). The mechanism of glucose transport through yeast cell membranes has attracted attention and also considered to be an important in vitro screening method for the hypoglycemic activity of crude extracts. The current study revealed that the extracts obtained from BPS4, BPS7, BPS17, BPR7, BPK9 and BPR10 showed facilitate glucose transport through yeast cells. The amount of glucose remaining in the medium after a certain period of time acts as a measure of glucose uptake by yeast cells. Slowing down the production and/or absorption of glucose is considered as an important strategy for managing diabetes.
The medicinal plants improves glucose uptake by translocation of GLUT4, which is confirmed by in vitro glucose models. The study reported that L6 and 3T3 cell lines are the most typical sources of cell models for studying glucose uptake and GLUT4 translocation (Das and Devi, 2015). Therefore, the current study used 3T3 cell lines to determine the glucose absorption activity of dichloromethane and ethyl acetate extracts of BPS17 and BPK9 respectively. The result revealed decreases in glucose concentration from 0th hour to 24th hour of observation period. The presence of bioactive molecules in the endophytic fungal isolates as the ability to induce the absorption of glucose at such low concentrations suggested that it could be a good scaffold as a starting point for the drug development (Jayant and Vijayakumar, 2021). It is evident that dichloromethane (D. eschscholtzii) and ethyl acetate (P. mangiferae) extracts were recognized as potent inhibitors of glucose diffusion, showing the highest inhibition percentage of glucose diffusion. Cytotoxic activity was exhibited by ethyl acetate extract of D. eschscholtzii against lung adinocarcinoma (A549) and mouse fibroblast (NIH3T3) cell lines. Cytotoxic effect ranges from 25.4 ± 0.3 to 92.7 ± 0.6% and 0.8 ± 0.1 to 6.9 ± 0.3% against A549 and NIH3T3 cell line respectively (Chutulo and Chalannavar, 2020). These findings on anti-diabetic activity of known and unknown species of endophytic fungi are in agreement with the previous study reports. In addition to the usual anti-diabetic drugs, endophytic fungi isolated from B. pinnatum are considered as an adjuvant in the treatment of diabetes.
Phenolic compounds, such as flavonoids and terpenoids, are the main antioxidant substances that eliminate free radicals and thus neutralize reactive oxygen species. Flavonoids use different mechanisms, such as inhibition of enzymes involved in the production of free radicals, thus preventing the development of oxidative stress (Shah et al., 2019). Complications of diabetes are predicted as a result of oxidative stress due to the formation of free radicals during the oxidation of glucose. Therefore, in order to avoid such complications, it is often recommended to use antioxidants along with anti-diabetic drugs (Jayant and Vijayakumar, 2021). In this study, the potent strains of endophytes, BPS17 and BPK9 showed the presence of flavonoids and terpenoids and these phytochemicals are responsible for the antioxidant activity shown by the crude extracts obtained, so this study supports the previous study reports.
The previous study reported that antioxidant activity was exhibited by D. eschscholtzii and Daldinia placentiformis isolated from Tridax procumbens (Misra et al., 2019). In this findings, 87.3 ± 0.8% inhibition of free radicals was exhibited by D. eschscholtzii. The anti-diabetic activity shown by ethanol extract of P. hababianum may be related to its powerful antioxidant activity and therefore it is effective in preventing oxidative and cellular damage of β-pancreatic cells. The endophyic fungi which showed good anti-diabetic activity also exhibited good antioxidant activity. (Ganesan et al., 2016). These findings are in direct correlation with the previous findings. Therefore, the endophytic fungi isolated from B. pinnatum acts as an anti-diabetic as well as antioxidant agents.
Bioprospecting of endophytic fungi for biologically active metabolites is beneficial because once isolated, they can be reproduce, safe and helps to preserve biodiversity. This is the first report on isolation of D. eschscholtzii and P. mangiferae from stem and root parts of B. pinnatum. Thus, endophytic D. eschscholtzii and P. mangiferae are considered as a potential source in anti-diabetic drug development process.
Conclusion
The dichloromethane and ethyl acetate extracts of D. eschscholtzii and P. mangiferae are considered as the potent strains of anti-diabetic and antioxidant effects.
Ethical Issue
The development, acquisition, authentication, cryopreservation, and transfer of cell lines between laboratories were followed according to the guidelines published in British Journal of Cancer, 2014.
Acknowledgement
The authors thank the Vellore Institute of Technology, Vellore for providing necessary lab facilities to carry out this research.
References
Ali IJ, Anyanwu OO, Chukwudulue UM, Chinaka CN, Ogbiko C, Awemu G, Ahomafor JE, Onyeloni SO, Okolo CC, Obidiegwu OC, Okoye FB. Pharmacological and phytochemical potentials of endophytic fungi from Nigerian medicinal plants: A review. GSC Biol Pharm Sci. 2023; 23: 20-28.
Aransiola EF, Daramola MO, Iwalewa EO, Seluwa AM, Olufowobi OO. Anti-diabetic effect of Bryophyllum pinnatum leaves. Int J Biotechnol Bioeng. 2014; 8: 89-93.
Bai Y, Yi P, Zhang S, Hu J, Pan H. Novel antioxidants and α-glycosidase and protein tyrosine phosphatise 1B inhibitors from an endophytic fungus Penicillium Brefeldianum F4a. J Fungi. 2021; 7: 1-10.
Baliyan S, Mukherjee R, Priyadarshini A, Vibhuti A, Gupta A, Pandey RP, Chang CM. Determination of antioxidants by DPPH radical scavenging activity and quantitative phytochemical analysis of Ficus religiosa. Molecules. 2022; 27: 1326.
Belayneh YM, Birru EM. Antidiabetic activities of hydromethanolic leaf extract of Calpurnia aurea (Ait.) Benth. Subspecies aurea (Fabaceae) in mice. Evid Based Complement Altern Med. 2018; 2018
Bhardwaj A, Sharma D, Jadon N, Agrawal PK. Antimicrobial and phytochemical screening of endophytic fungi isolated from spikes of Pinus roxburghii. Arch Clin Microbiol. 2015; 6: 1-9.
Chutulo EC, Chalannavar RK. Daldinia eschscholtzii: An endophytic fungi isolated from Psidium guajava as an alternative source of bioactive secondary metabolites. Asian J Mycol. 2020; 3: 376-98.
Daniel IE, Akpan EI, Utam EC. Phytochemical evaluation, antioxidant and antimicrobial activities of various extracts from leaves and stems of Bryophyllum pinnatum. Nepal J Biotechnol. 2020; 8: 17-28.
Das MS, Devi G. In vitro cytotoxicity and glucose uptake activity of fruits of Terminalia bellirica in vero, L-6 and 3T3 cell lines. J Appl Pharm Sci. 2015; 5: 92-95.
Effah-Yeboah E, Dartey E, Asare EA, Abraham JD, Kagya-Agyemang JK, Balali GI, Aboagye V. Effect of Kalanchoe crenata extract on renal and liver impairment, dyslipedemia and glycemia in streptozotocin induced diabetic rats. Annu Res Rev Biol. 2021; 36: 109-25.
Ezeagu CU, Elijah JP, Nwodo OF. Antidiabetic potential of ethanol leaf extract of Bryophyllum pinnatum on alloxan-induced diabetic rats and their haematological profiles. Afr J Pharm Pharmacol. 2017; 11: 526-33.
Ferdous KJ, Sohrab MH, Begum MN, Islam MR, Mazid MA. Morphological and molecular identification of endophytic fungi isolated from Zingiber officinale rocs. Bangladesh J Plant Taxon. 2022; 29: 361-71.
Fernandes JM, Cunha LM, Azevedo EP, Lourenço EM, Fernandes-Pedrosa MF, Zucolotto SM. Kalanchoe laciniata and Bryophyllum pinnatum: An updated review about ethnopharmacology, phytochemistry, pharmacology and toxicology. Braz J Pharm. 2019: 29: 529-58.
Gulyamova TG, Nasmetova SM, Ruzieva DM, Mukhammedov II, Kadyrova GK, Karimova FA. Phytochemical analysis of α-amylase inhibiting secondary metabolites of endophytic Penicillium brevicaule alba Thom. Eur J Mol Clin Med. 2020; 7: 830-36.
Gupta S, Adak S, Rajak RC, Banerjee R. In vitro efficacy of Bryophyllum pinnatum leaf extracts as potent therapeutics. Prep Biochem Biotechnol. 2015; 46: 489-94.
Gurnani N, Gupta M, Mehta D, Mehta BK. Chemical composition, total phenolic and flavonoid contents and in vitro antimicrobial and antioxidant activities of crude extracts from red chilli seeds (Capsicum frutescens L.). J Taibah Univ Sci. 2016; 10: 462-70.
Hamzah TN, Lee SY, Hidayat A, Terhem R, Faridah-Hanum I, Mohamed R. Diversity and characterization of endophytic fungi isolated from the tropical mangrove species, Rhizophora mucronata and identification of potential antagonists against the soil-borne fungus, Fusarium solani. Front Microbiol. 2018; 9: 1-17.
Jayamol MA, Ashley R, Nithya J, Shijina S. Effect of combination of aqueous leaf extracts of Psidium guajava Linn and Moringa oleifera Lam on diabetes mellitus. Int J Pharm Pharm Sci. 2020; 12: 79-82.
Jayant KK, Vijayakumar BS. In-vitro antioxidant and anti-diabetic potential of endophytic fungi associated with Ficus religiosa. Ital J Mycol. 2021; 50: 10-20.
Joseph B, Jini D. Antidiabetic effects of Momordica charantia (bitter melon) and its medicinal potency. Asian Pac J Trop Dis. 2013; 3: 93-102.
Kaur A. Evaluation of anti-diabetic and antioxidant potential of endophytic fungi isolated from medicinal plants. Int J Green Pharm. 2018; 12: 1-14.
Kumar TS, Muthusamy P, Radha R, Ilango K. Formulation and evaluation of in vitro anti-diabetic polyherbal tablets from some traditional used herbs. J Phytopharm. 2021; 10: 173-79.
Kumari M, Silva ID. Alpha-amylase inhibition activity of endophytic fungi isolated from leaves of Artocarpus heterophyllus. Int j Sci Res. 2018; 7: 731-34.
Mbachu KA, Ibok MG, Adeniyi-Akee MA, Ajala OE. Chemical compositions and antioxidant activity of leaf and stem essential oil of Bryophyllum pinnatum. GSC Biol Pharm Sci. 2019; 09: 057-64.
Mishra R, Kushveer JS, Khan MK, Sarma VV. Evaluation of antioxidant potential, DNA damage protection and anti-cancer activities of three endophytic fungi associated with selected medicinal plants. Int J Pharm Biol Sci. 2019; 9: 1174-84.
Naveen KV, Saravanakumar K, Sathiyaseelan A, Wang MH. Comparative analysis of the antioxidant, anti-diabetic, antibacterial, cytoprotective potential and metabolite profile of two endophytic Penicillium spp. Antioxidants 2023; 12: 1-22.
Ogbonnia SO, Odimegwu JI, Enwuru VN. Evaluation of hypoglycaemic and hypolipidaemic effects of aqueous ethanolic extracts of Treculia africana Decne and Bryophyllum pinnatum Lam. and their mixture on streptozotocin (STZ)-induced diabetic rats. Afr J Biotechnol. 2008; 7: 2535-39.
Ojewole JAO. Antinociceptive, anti-inflammatory and anti-diabetic effects of Bryophyllum pinnatum (Crassulaceae) leaf aqueous extract. J Ethnopharmacol. 2005: 99: 13-19.
Ojo OA, Ojo AB, Ajiboye BO, Olaiya O, Akawa A, Olaoye O, Anifowose OO, Idowu O, Olasehinde O, Obafemi T, Awe J. Inhibitory effect of Bryophyllum pinnatum (Lam.) Oken leaf extract and their fractions on α-amylase, α-glucosidase and cholinesterase enzyme. Pharmacogn J. 2018; 10: 497-506.
Okonkwo-Uzor NJ, Okezie UM, Eze PM, Ikegbune C, Okoye FB, Esimone CO. In vitro antimicrobial activity of secondary metabolites of an endophytic Aspergillus brunneoviolaceus isolated from Bryophyllum pinnatum (Lam.) Oken. J Clin Basic Res. 2022; 2: 249-54.
Paul S, Majumdar M. In vitro antidiabetic propensities, phytochemical analysis and mechanism of action of commercial antidiabetic polyherbal formulation Mehon. Proceedings. 2021; 79: 1-8.
Pavithra N, Sathish L, Babu N, Venkatarathanamma V, Pushpalatha H, Reddy GB, Ananda K. Evaluation of α-amylase, α-glucosidase and aldose reductase inhibitors in ethyl acetate extracts of endophytic fungi isolated from anti-diabetic medicinal plants. Int J Pharm Sci Res. 2014; 5: 5334-41.
Pottathil S, Nain P, Morsy MA, Kaur J, Al-Dhubiab BE, Jaiswal S, Nair AB. Mechanisms of antidiabetic activity of methanolic extract of Punica granatum leaves in nicotinamide/streptozotocin-induced type-2 diabetes in rats. Plants 2020; 9: 1-15.
Rustamova N, Gao Y, Zhang Y, Yili A. Biological activity of endophytic fungi from the roots of the medicinal plant Vernonia anthelmintica. Microorganisms 2020; 8: 586.
Sagbo IJ, van de Venter M, Koekemoer T, Bradley G. In vitro antidiabetic activity and mechanism of action of Brachylaena elliptica (Thunb.) DC. Evid Based Complement Altern Med. 2018; 2018.
Shah MAR, Khan RA, Ahmed M. Anti-diabetic activity of Iphiona aucheri leaf extract. Bangladesh J Pharmacol. 2020; 15: 99-109.
Shah MAR, Rahmat AK, Mushtaq A. Phytochemical analysis, cytotoxic, antioxidant and anti-diabetic activities of the aerial parts of Sorghum halepense. Bangladesh J Pharmacol. 2019; 14: 144-51.
Shankar S, Settu S, Segaran G, Sundar RD, Ravi L. Phytochemical constituents of Dracaena mahatma leaves and their antibacterial, antioxidant and anti-inflammatory significance. Biotechnol Res Innov. 2018; 2: 1-8.
Shettar AK, Vedamurthy AB. Evaluation of anti-diabetic potential of Kandelia candel and Rhizophora apiculta: An in vitro approach. Int J Pharm Sci Res. 2017; 8: 2551-59.
Shoba S, Sathiavelu M. Biological activities of endophytic fungus Cochliobolus sp.AL24 isolated from Aerva lanata L. Indian J Pharm Educ Res. 2018; 52: 277-83.
Singh B, Kaur A. Antidiabetic potential of a peptide isolated endophytic fungi Aspergillus awamori. J Appl Microbiol. 2016; 120: 301-11.
Sofa F, Akhmad D, Megawati M, Muhammad H. Isolation and identification of quercetin derivatives and their α-glucosidase inhibitory activities from Bryophyllum pinnatum. Res J Chem Environ. 2018; 22: 114-19.
Sowemimo AA, Edrada-Ebel R, Ebel R, Proksch P, Omobuwajo OR, Adesanya SA. Major constituents of the predominant endophytic fungi from the Nigerian plants Bryophyllum pinnatum, Morinda lucida and Jathropha gossypiifolia. Nat Prod Commu. 2008; 3: 1934578X0800300802.
Tejaswini HK, Jayashankar M, Saeed MA. Screening of biological effectiveness of endophytic fungi associated with Plectranthus amboinicus: A focus on Aspergillus terreus. Int J Pharm Sci Rev Res. 2021; 69: 24-31.
Tiwari P, Nathiya R, Mahalingam G. Antidiabetic activity of endophytic fungi isolated from Ficus religiosa. Asian J Pharm Clin Res. 2017; 10: 59-61.
Venkateswarulu N, Shameer S, Bramhachari PV, Thaslim basha Sk, Nagaraju C, Vijaya T. Isolation and characterization of plumbagin (5-hydroxyl-2-methylnaptalene-1,4di-one) producing endophytic fungi Cladosporium delicatulum from endemic medicinal plants. Biotechnol Rep. 2018; 20.
Vidyasagar GM, Naaz SQ, Ahmed LMD, Babanagre S, Sangeeta MK, Ambika V. Penicillium citrinum Thom: A potential antihyperglycemic endophyte from Gymnema sylvestre. Indian J Nat Prod Resour. 2021; 12; 557-63.
White TJ, Bruns TD, Lee SB, Taylor JW. Amplification and direct sequencing of fungal ribosomal RNA genes for phylogenetics. 1990, pp 315-22.
Wutthiwong N, Suthiphasilp V, Pintatum A, Suwannarach N, Kumla J, Lumyong S, Maneerat T, Charoensup R, Cheenpracha S, Limtharakul T, Pyne SG. Daldiniaeschsone A, a rare tricyclic polyketide having a chromone unit fused to a δ-lactone and its symmetrical biphenyl dimer, daldiniaeschsone B, from an endophytic fungus Daldinia eschscholtzii SDBR-CMUNKC745. J Fungi. 2021; 7: 1-12.
Xiao F, Xu T, Lu B, Liu R. Guidelines for antioxidant assays for food components. Food Frontiers. 2020; 1: 60-69.
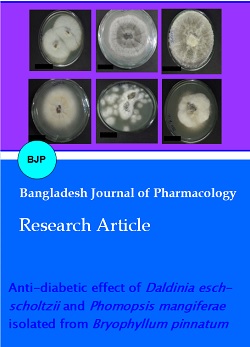