15,16-Dihydrotanshinone I inhibits the proliferation of MV4-11 by means of apoptosis via antagonizing FLT3-ITD/STAT5/Mcl-1 pathway
Abstract
Till now, the medicines approved for acute myeloid leukemia with internal tandem duplication mutation of FMS-like tyrosine kinase 3 (FLT3-ITD) display not ideal efficacy. This study aimed to evaluate the effects of 15,16-dihydrotanshinone I on FLT3-ITD acute myeloid leukemia cells. The inhibitory effect of this compound against MV4-11 was determined using CCK-8 assay. Western blotting detecting caspase-3, PARP, and annexin V-APC/7-AAD was carried out. Activation of FLT3, STAT5, and Mcl-1 expression was analyzed by western blotting. The results showed that MV4-11 was sensitive toward dihydrotanshinone I in a dose-dependent manner (p<0.05). MV4-11 apoptosis was induced notably after dihydrotanshinone I treatment. Western blotting revealed suppressed activation of FLT3, STAT5 and decreased Mcl-1 (p<0.05). This study suggests that dihydrotanshinone I inhibits MV4-11 proliferation by apoptosis via antagonizing FLT3-ITD/STAT5/Mcl-1 pathway, which might provide a novel therapy for acute myeloid leukemia.
Introduction
Acute myeloid leukemia is a severe hematologic disease in which somatic mutation of internal tandem duplication (ITD) in the FMS-like tyrosine kinase 3 (FLT3) dominate nearly 30% of primaryly diagnosed acute myeloid leukemia cases (Hogan et al., 2020). FLT3 belongs to the class III receptor tyrosine kinase family, which comprises c-KIT, macrophage colony-stimulating factor (M-CSF), and platelet-derived growth factor (PDGF) receptors (Scheijen and Griffin, 2002). Physiologically, its activation depends on the FLT3 ligand. However, ITD mutation in the juxtamembrane domain of FLT3 is considered to result in constitutive auto-phosphorylation of itself, which gives rise to excessive downstream signaling pathways. Among them, FLT3-ITD/signal transducers and activators of transcription (STAT5)/anti-apoptotic molecules signaling are particularly important (Carter et al., 2020).
In acute myeloid leukemia blast, the sustained self-activation of FLT3-ITD reactivates STAT5 by phosphorylation, which transactivates some anti-apoptosis genes, for instance, myeloid cell leukemia-1 (Mcl-1), B-cell lymphoma-2 (Bcl-2), Pim (Mizuki et al., 2003; Singh Mali et al., 2021; Takahashi et al., 2004; Yoshimoto et al., 2009). Moreover, FLT3-ITD is deemed as a hallmark for the relapse, drug resistance, and other adverse prognosis of clinical acute myeloid leukemia (Müller and Schmidt-Arras, 2020). Therefore, as the prime culprit, FLT3-ITD attracts much interest from some investigators. However, FLT3 inhibitors approved for FLT3-ITD acute myeloid leukemia therapy in clinical practice are testified less ideal nowadays (Burchert, 2021). Therefore, the development of other effective agents is still the focus of acute myeloid leukemia therapy.
15,16-Dihydrotanshinone I, a component of Salvia miltiorrhiza (danshen), is reported effective in handling some preclinical diseases such as type II diabetes (Jeong et al., 2017), infantile hemangioma (Duan et al., 2021), and ovarian cancer (Wang et al., 2020). As for the mechanisms, these studies indicate various actions this component could exert, depending on the context of experimented cells. Dihydrotanshinone I induces HL-60, an acute myeloid leukemia cell line harboring wild-type FLT3 (FLT3-WT), apoptosis in vitro, and inhibits its growth in vivo (Liu et al., 2015). However, its effects on FLT3-ITD acute myeloid leukemia haven’t been reported elsewhere.
Based on these, this study is designed to examine the effect of dihydrotanshinone I toward MV4-11, an FLT3-ITD acute myeloid leukemia cell line. The results demonstrate that dihydrotanshinone I inhibits the proliferation of FLT3-ITD acute myeloid leukemia cells by reversal of anti-apoptosis induced by FLT3-ITD/STAT5/Mcl-1 pathway.
Materials and Methods
Cell culture
MV4-11 was kindly provided by the stem cell bank, Chinese Academy of Science (Shanghai, China) and maintained in RPMI-1640 (Gibco, USA) supplemented with 10%fetal bovine serum (FBS) and 1%penicillin-streptomycin (v/v) at 37°C in a humidified atmosphere containing 5%CO2.
Reagents and antibodies
The CCK-8 (#N31213) and annexin V-APC/7-AAD apoptosis kits (#E-CK-A218) were purchased from TransGen Biotech (China) and Elabscience Biotech Co., Ltd. (China), respectively. Primary rabbit antibodies against cleaved caspase-3 (#9661S), PARP (#9532), FLT3 (#3462), p-FLT3 (#3464), STAT5 (#94205S), p-STAT5a/b (#4322S), Mcl-1 (#39224S) were all purchased from CST (USA). Rabbit anti-β-actin (#bsm-33036M), anti-α-tublin (#14555-1-AP) were purchased from Bioss Biotech Co., LTD (China) and Proteintech (China), respectively.
Cell viability
CCK-8 experiment was performed to assess the sensitivity of MV4-11 towards dihydrotanshinone I (#D0947, Sigma-Aldrich, USA). In brief, after washing cells twice with ice-PBS (pH 7.2-7.6), 3.0-4.0 × 104 cells in 90 μL RPMI-1640 containing 0.5% heat-inactivated FBS were seeded into 96-well microtiter plates, followed by the addition of 10 μL RPMI-1640 or dihydrotanshinone I at different concentrations (25-200 μM), and incubated at 37°C, 5%CO2 for 15 hours, followed by adding CCK-8 solution (10 μL). After 3 hours, the optical density at 450 nm wavelength (OD450) was determined using an ELISA scanner.
Annexin V/7-AAD analysis
To evaluate the pro-apoptotic effect of dihydrotan-shinone I on MV4-11 cells, APC annexin V apoptosis detection kit with 7-AAD was used. This detection kit can detect the rate of necrotic, early apoptotic, and late apoptotic cells in the cell suspension. For this analysis, Cells were seeded at a density of 3.5 × 105 per well in 24-well plates and treated with RPMI-1640 or dihydrotanshinone I (10 μM) for 5 hours at 37°C, 5%CO2. After that, cells were washed 2 times with ice-cold PBS (pH 7.2-7.6). The cells were resuspended with annexin-V-binding buffer and after the cells were transferred to a flow cytometry tube, 5 µL of annexin V-APC and 10 µL of 7-AAD viability staining solution were added to 100 µL of cell suspension. Finally, the apoptotic effect of dihydrotanshinone I was measured by flow cytometry using FACSCanto (BD Biosciences).
Box I: Western Blotting
Western blotting is used for detection and characterization of target proteins. It is based on the principle of immunochromatography where proteins are separated into polyacrylamide gel according to their molecular weight.
Requirements
Primary antibodies against cleaved caspase-3 (#9661S; 1:1000; CST), PARP (#9532; 1:1000;CST), FLT3 (#3462; 1:1000; CST), phospho-FLT3 (#3464; 1:1000; CST), STAT5 (#94205S; 1:1000; CST), p-STAT5a/b (#4322S; 1:1000; CST), Mcl-1 (#39224S; 1:1000; CST), β-actin (#bsm-33036M; 1:5000; Bioss Biotech) or α-tublin (#14555-1-A; 1:50000; Proteintech); HRP-conjugated goat anti-rabbit secondary antibodies (#ab181602; 1:10000; ZSGB-BIO); RIPA lysis buffer (Solarbio, China); SDS-PAGE (10% gel); PVDF membranes (Millipore, Merck)
Procedure
Step 1: Cells were seeded at a density of 1.5 × 106 per well in 6-well plates and treated with RPMI-1640 or 15,16-dihydrotanshinone I (10 μM) for 5 hours at 37°C, 5%CO2
Step 2: For protein extraction, the cells were centrifuged (300 × g, 5 min)
Step 3: The pellets were washed once with ice-cold PBS (pH 7.2-7.6), extracted with 100 μL RIPA lysis buffer containing a cocktail of protease/phosphatase inhibitor (1 mM), and quantified using BCA protein assay kits according to the manufacturer’s instructions
Step 4: The proteins were separated using sodium dodecyl sulfate-polyacrylamide gel electrophoresis (10%) and transferred onto polyvinylidene fluoride membranes (0.45 μm), which were blocked with 5% fat-free milk and then incubated with primary antibodies (anti-cleaved caspase-3, 1:1000; anti-PARP, 1:1000; anti-FLT3, 1:1000; anti-p-FLT3, 1:1000; anti-STAT5, 1:1000; anti-p-STAT5a/b, 1:1000; anti-Mcl-1, 1:1000; anti-β-actin, 1:5000; anti-α-tublin, 1:50000) at 4°C overnight
Step 5: After washing 5 times with tris-buffered saline and tween-20 (0.1%), the membranes were probed with horseradish peroxidase-conjugated secondary antibodies (goat anti-rabbit IgG, 1:10000) for 1 hour at room temperature
Step 6: Finally, the protein bands were visualised using an enhanced chemiluminescence detection kit (NCM Biotech, China) and developed in a gel imager (VILBER/FUSION FX, France).
References (Video)
Eslami and Lujan, 2010; Lim et al., 2021; Shen et al., 2021
Statistical analysis
All experiments were performed in triplicate and all data were presented as mean values ± standard deviation (SD). GraphPad Prism software ver. 7.0 (GraphPad Software, Inc., USA) was used for all statistical analyses. The differences between groups were evaluated with Student’s t-test and one-way ANOVA. p<0.05 value was considered statistically significant.
Results
Proliferation of MV4-11 cells
In a study, 15,16-dihydrotanshinone I exhibited anti-tumor activity in the human acute myeloid leukemia cell line HL-60, which harbors FLT3-WT. Thus, CCK-8 assay was used to evaluate the efficacy of this component in MV4-11, an acute myeloid leukemia cell line with FLT3-ITD mutation. Dihydrotanshinone I displayed markedly inhibitory activity towards MV4-11 in a dose-dependent manner (p<0.05) (Figure 1). It is worth noting that when the concentration of dihydrotanshinone I was ≥10 μM, the inhibitory effect was more significant. When the incubation was prolonged to 24 hours, dihydrotanshinone I (10 μM) nearly cleared the cells completely (data not shown).
Figure 1: Sensitivity of MV4-11 cells towards dihydrtanshinone I. MV4-11 suspension (3-4 × 104/90 μL/well) was co-incubated with serially diluted dihydrotanshinone I (10 μL) under 37℃, 5%CO2 for 15 hours, followed by detection using CCK-8 assay. ap˂0.05, bp<0.01
MV4-11 apoptosis after 15,16-dihydrotanshinone I treatment
To assess whether the inhibitory mechanism of dihydrotanshinone I on MV4-11 cells was associated with apoptosis, the activation of both caspase-3 and PARP was analyzed by western blotting. As shown in Figure 2A, B, C, these two apoptosis-related molecules were activated significantly compared to the control group (p<0.05). To verify the pro-apoptosis effect dihydrotanshinone I induced on MV4-11 cells, annexin V-APC/7-AAD analysis was further performed. It was determined that cellular apoptosis (early apoptosis) was significantly increased in cells treated with dihydrotanshinone I compared with that of negative control (Figure 2D, p<0.05).
Figure 2: MV4-11 apoptosis induced by dihydrotanshinone I (DHTS). MV4-11 (1.5 × 106) suspended in RPMI-1640 (0.5%FBS) were treated with RPMI-1640 (negative control) or DHTS (10 μM) at 37℃, 5%CO2 for 5 hours in 6-well plates, followed by protein extraction and subsequent cleaved caspase-3, PARP detection by western blotting (A). B: the gray density comparison of cleaved caspase-3; C: the gray density comparison of PARP. MV4-11 (3.5 × 105) suspended in 0.5%FBS RPMI-1640 were treated with RPMI-1640 (negative control) or DHTS (10 μM) at 37℃, 5%CO2 for 5 hours in 24-well plates, followed by FACS analysis after using an annexin V-APC/7-AAD staining kit (D). ap˂0.05, cp<0.001
FLT3-ITD/STAT5/Mcl-1 pathway in MV4-11 cells
Because of the characterized anti-apoptosis activity of FLT3-ITD acute myeloid leukemia cells via FLT3-ITD/STAT5/anti-apoptotic molecules pathway, it was fur-ther wondered whether the observed pro-apoptosis effects 15,16-dihydrotanshinone I induced on MV4-11 cells involved influencing this abnormal signaling. The western blotting results demonstrated that phosphorylation of both FLT3-ITD (Figure 3A) and STAT5 (Figure 3B) were suppressed obviously when MV4-11 was treated by dihydrotanshinone I for 5 hours (p<0.05). As a downstream molecule of STAT5, Mcl-1 expression was also reduced in MV4-11 cells after co-incubation with dihydrotanshinone I (Figure 3C).
Figure 3: Dihydrotanshinone I (DHTS) antagonized FLT3-ITD/STAT5/Mcl-1 pathway in MV4-11 cells. The acute myeloid leukemia cell line MV4-11 (1.5 × 106) suspended in 0.5%FBS RPMI-1640 were treated with RPMI-1640 (negative control) or DHTS (10 μM) at 37℃, 5%CO2 for 5 hours in 6-well plates, after which cell lysates were subjected to SDS-PAGE and western blotting using specific antibodies described in “Materials and Methods†section. α-Tublin was used as sample loading control. ap˂0.05, bp<0.01, cp<0.001
Discussion
These years, many studies have been undertaken to develop drugs for the treatment of acute myeloid leukemia, particularly FLT3-ITD acute myeloid leukemia. 15,16-Dihydrotanshinone I, a component extracted from Salvia miltiorrhiza Bunge (Tanshen), is testified effectively in suppressing human acute myeloid leukemia cell line HL-60 which harbors FLT3-WT (Liu et al., 2015). Whether this effect similarly exists in FLT3-ITD acute myeloid leukemia cells remains unknown. The results of the present work coherently suggest that dihydrotanshinone I inhibits FLT3-ITD acute myeloid leukemia cells via apoptosis. The potential mechanisms involved in this effect might be associated with antagonizing the anti-apoptosis induced by FLT3-ITD/STAT5/Mcl-1 pathway.
In acute myeloid leukemia cells, FLT3-ITD mutation is considered to constitutively activate this mutant tyrosine kinase through self-dimerization and ensuing phosphorylation (Kiyoi et al., 1998). This ligand-independent FLT3 activation induces its downstream signalings, which results in diminished apoptosis and uncontrolled cell proliferation (Takahashi, 2011). Therefore, anti-apoptosis antagonism is believed to be a promising direction for acute myeloid leukemia therapy. In this study, the proliferation of MV4-11 was inhibited by dihydrotanshinone I in a dose-dependent manner. Because of the anti-apoptosis character for FLT3-ITD acute myeloid leukemia cells, caspase-3/PARP western blot and FACS were used to evaluate the apoptosis of MV4-11 after treatment by dihydrotanshinone I. As shown in Figure 2, under concentrations of 10 μM, dihydrotanshinone I induced significant apoptosis in contrast with negative control. This phenomenon was in line with that observed in another study (Liu et al., 2015), even though the acute myeloid leukemia cell line they used was HL-60 which bears FLT3-WT. Interestingly, in a preliminary work, HL-60 showed less sensitivity towards dihydrotanshinone I than MV4-11 did (data not shown). It appears to imply that FLT3-WT and FLT3-ITD acute myeloid leukemia cells are sensitive toward dihydrotanshinone I, particularly the latter. The mechanism mediating its inhibitory effect probably ascribes to the pro-apoptosis function of dihydrotanshinone I. However, the exact signaling pathways require further investigation.
In FLT3-ITD acute myeloid leukemia cells, it is well known that the pronounced pro-survival feature is mainly attributed to the aberrant anti-apoptosis signaling pathway FLT3-ITD/STAT5/anti-apoptotic molecules (Debierre-Grockiego, 2004). Therefore, in the following experiments, the condition of this pathway in MV4-11cells under dihydrotanshinone I treatment. Intriguingly, the results turned out that after 5 hours of treatment with dihydrotanshinone I markedly inhibited the activation of this pathway, evidenced by reduced phosphorylation of both FLT3 and STAT5. In addition, the decreased expression of Mcl-1 which is a target gene of STAT5, was also detected. Combined with the descriptions above, it indicates that dihydrotanshinone I probably exerts its inhibitory activity against FLT3-ITD acute myeloid leukemia cells by apoptosis through antagonizing the anti-apoptosis induced by FLT3-ITD/STAT5/Mcl-1 signaling. As a nuclear transcription factor, STAT5 activation relies on the phosphorylation by some protein tyrosine kinases (PTK), i.e., FLT3-ITD (Choudhary et al., 2007). However, as to how FLT3-ITD activates STAT5, there are some different opinions. Some studies demonstrate that FLT3-ITD activates STAT5 directly, while a few researchers suggest that STAT5 may be activated by FLT3-ITD indirectly via Janus kinase 2 (JAK2) (Debierre-Grockiego, 2004). After STAT5 activation, some genes coding anti-apoptotic molecules, for example, Mcl-1, Bcl-2, Pim-1, will be transactivated, which results in acute myeloid leukemia cell survival and proliferation (Mizuki et al., 2003; Singh Mali et al., 2021; Yoshimoto et al., 2009). Among these terminal molecules, Mcl-1 is a classical delegate dependent of STAT5 (Yoshimoto et al., 2009). It belongs to Bcl-2 family, and boycotts apoptosis through binding to the pro-apoptotic Bcl-2 proteins (Shahar and Larisch, 2020). Mcl-1 overexpression is frequently observed in many tumor types and is closely associated with tumorigenesis, poor prognosis, and drug resistance (Hird and Tron, 2019). However, as another anti-apoptotic downstream molecule of STAT5, the reduced expression of Bcl-2 wasn’t determined in the present experiments.
As to the underlying mechanism (s) for the antagonizing effects of dihydrotanshinone I towards FLT3-ITD/STAT5/Mcl-1 pathway in MV4-11 cells, it was postulated that human antigen R (HuR), a nuclear mRNA-binding protein (RBP), presumably plays an important role. As an RBP, HuR orchestrates the stabilization and translation of mRNAs, which is pivotal for inflammation and tumor progression (Kang et al., 2020). Some studies observed the binding effect of HuR towards the mRNA of Bcl-2, Mcl-1, and other anti-apoptotic molecules (Srikantan and Gorospe, 2012). More importantly, dihydrotanshinone I interferes with the RNA-binding action of HuR, which affects its post-transcriptional regulatory activity (D'Agostino et al., 2015; Lal et al., 2017). Whether this mechanism can explain the results of this study needs further investigation.
Conclusion
This study revealed the inhibitory effect of 15,16-dihydrotanshinone I towards MV4-11, a classical cell line of FLT3-ITD acute myeloid leukemia, through apoptosis. The underlying mechanisms involved in this effect was associated with antagonising the anti-apoptosis induced by FLT3-ITD/STAT5/Mcl-1 pathway, which provides a novel selection for acute myeloid leukemia therapy.
Acknowledgements
Authors sincerely thank Mr. Ping Ye and Mr. Yulan Liu from the Nanchang University Affiliated Ganzhou Hospital for their arduous work of FACS analysis in this study.
References
Burchert A. Maintenance therapy for FLT3-ITD-mutated acute myeloid leukemia. Haematologica 2021; 106: 664-70.
Carter JL, Hege K, Yang J, Kalpage HA, Su Y, Edwards H, Hüttemann M, Taub JW, Ge Y. Targeting multiple signaling pathways: the new approach to acute myeloid leukemia therapy. Signal Transduct Target Ther. 2020; 5: 288.
Choudhary C, Brandts C, Schwable J, Tickenbrock L, Sargin B, Ueker A, Böhmer FD, Berdel WE, Müller-Tidow C, Serve H. Activation mechanisms of STAT5 by oncogenic Flt3-ITD. Blood 2007; 110: 370-4.
D'Agostino VG, Lal P, Mantelli B, Tiedje C, Zucal C, Thongon N, Gaestel M, Latorre E, Marinelli L, Seneci P, Amadio M, Provenzani A. Dihydrotanshinone-I interferes with the RNA-binding activity of HuR affecting its post-transcriptional function. Sci Rep. 2015; 5: 16478.
Debierre-Grockiego F. Anti-apoptotic role of STAT5 in haematopoietic cells and in the pathogenesis of malignancies. Apoptosis 2004; 9: 717-28.
Duan P, Huang Y, Chen K, Cheng C, Wu Z, Wu Y. 15,16-dihydrotanshinone I inhibits EOMA cells proliferation by interfering in posttranscriptional processing of hypoxia-inducible factor 1. Int J Med Sci. 2021; 18: 3214-23.
Eslami A, Lujan J. Western blotting: Sample preparation to detection. JoVE. 2010; 44: e2359.
Hird AW, Tron AE. Recent advances in the development of Mcl-1 inhibitors for cancer therapy. Pharmacol Ther. 2019; 198: 59-67.
Hogan FL, Williams V, Knapper S. FLT3 Inhibition in acute myeloid leukaemia: Current knowledge and future prospects. Curr Cancer Drug Targets. 2020; 20: 513-31.
Jeong H, Koh A, Lee J, Park D, Lee JO, Lee MN, Jo KJ, Tran HNK, Kim E, Min BS, Kim HS, Berggren PO, Ryu SH. Inhibition of C1-Ten PTPase activity reduces insulin resistance through IRS-1 and AMPK pathways. Sci Rep. 2017; 7: 17777.
Kang D, Lee Y, Lee JS. RNA-Binding proteins in cancer: Functional and therapeutic perspectives. Cancers (Basel). 2020; 12: E2699.
Kiyoi H, Towatari M, Yokota S, Hamaguchi M, Ohno R, Saito H, Naoe T. Internal tandem duplication of the FLT3 gene is a novel modality of elongation mutation which causes constitutive activation of the product. Leukemia 1998; 12: 1333-7.
Lal P, Cerofolini L, D'Agostino VG, Zucal C, Fuccio C, Bonomo I, Dassi E, Giuntini S, Di Maio D, Vishwakarma V, Preet R, Williams SN, Fairlamb MS, Munk R, Lehrmann E, Abdelmohsen K, Elezgarai SR, Luchinat C, Novellino E, Quattrone A, Biasini E, Manzoni L, Gorospe M, Dixon DA, Seneci P, Marinelli L, Fragai M, Provenzani A. Regulation of HuR structure and function by dihydrotanshinone-I. Nucleic Acids Res. 2017; 45: 9514-27.
Lim HS, Jang Y, Moon BC, Park G. NF-κB signaling contributes to the inhibitory effects of Bombyx batryticatus on neuroinflammation caused by MPTP toxicity. Bangladesh J Pharmacol. 2021; 16: 96-102.
Liu JJ, Wu HH, Chen TH, Leung W, Liang YC. 15,16-Dihydrotanshinone I from the functional food Salvia miltiorrhiza exhibits anticancer activity in human HL-60 leukemia cells: In vitro and in vivo studies. Int J Mol Sci. 2015; 16: 19387-400.
Mizuki M, Schwable J, Steur C, Choudhary C, Agrawal S, Sargin B, Steffen B, Matsumura I, Kanakura Y, Böhmer FD, Müller-Tidow C, Berdel WE, Serve H. Suppression of myeloid transcription factors and induction of STAT response genes by AML-specific Flt3 mutations. Blood 2003; 101: 3164-73.
Müller JP, Schmidt-Arras D. Novel approaches to target mutant FLT3 leukaemia. Cancers (Basel). 2020; 12: E2806.
Scheijen B, Griffin JD. Tyrosine kinase oncogenes in normal hematopoiesis and hematological disease. Oncogene 2002; 21: 3314-33.
Shahar N, Larisch S. Inhibiting the inhibitors: Targeting anti-apoptotic proteins in cancer and therapy resistance. Drug Resist Updat. 2020; 52: 100712.
Shen S, Hou Y, Wu Y. Tongxinluo preserves the renal function in diabetic kidney disease via protecting the HK-2 cells from the patterns of programmed cell death. Bangladesh J Pharmacol. 2021; 16: 52-64.
Singh Mali R, Zhang Q, DeFilippis RA, Cavazos A, Kuruvilla VM, Raman J, Mody V, Choo EF, Dail M, Shah NP, Konopleva M, Sampath D, Lasater EA. Venetoclax combines synergistically with FLT3 inhibition to effectively target leukemic cells in FLT3-ITD+ acute myeloid leukemia models. Haematologica 2021; 106: 1034-46.
Srikantan S, Gorospe M. HuR function in disease. Front Biosci (Landmark Ed). 2012; 17: 189-205.
Takahashi S, Harigae H, Kaku M, Sasaki T, Licht JD. Flt3 mutation activates p21WAF1/CIP1 gene expression through the action of STAT5. Biochem Biophys Res Commun. 2004; 316: 85-92.
Takahashi S. Downstream molecular pathways of FLT3 in the pathogenesis of acute myeloid leukemia: Biology and therapeutic implications. J Hematol Oncol. 2011; 4: 13.
Wang X, Xu X, Jiang G, Zhang C, Liu L, Kang J, Wang J, Owusu L, Zhou L, Zhang L, Li W. Dihydrotanshinone I inhibits ovarian cancer cell proliferation and migration by transcriptional repression of PIK3CA gene. J Cell Mol Med. 2020; 24: 11177-87.
Yoshimoto G, Miyamoto T, Jabbarzadeh-Tabrizi S, Iino T, Rocnik JL, Kikushige Y, Mori Y, Shima T, Iwasaki H, Takenaka K, Nagafuji K, Mizuno S, Niiro H, Gilliland GD, Akashi K. FLT3-ITD up-regulates MCL-1 to promote survival of stem cells in acute myeloid leukemia via FLT3-ITD-specific STAT5 activation. Blood 2009; 114: 5034-43.
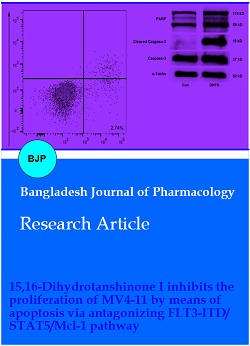